What is carbon capture, usage and storage (CCUS) and what role can it play in tackling climate change?
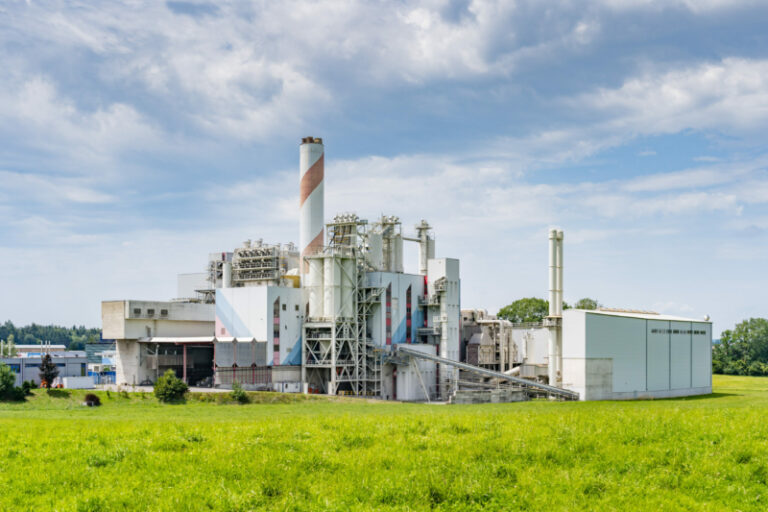
What is carbon capture, usage and storage (CCUS)?
CCUS refers to a suite of technologies that enable the mitigation of carbon dioxide (CO2) emissions from large point sources such as power plants, refineries and other industrial facilities, or the removal of existing CO2 from the atmosphere.
CCUS is expected to play a crucial role in meeting global climate targets. Leading organisations including the International Energy Agency (IEA), International Renewable Energy Agency (IRENA), Intergovernmental Panel on Climate Change (IPCC) and Bloomberg New Energy Finance (BNEF) have all produced long-term energy outlooks that rely on a rapid expansion of CCUS in order to limit global temperature rise to 1.5°C.
How does CCUS work?
A CCUS application consists of three stages: capture, transport and storage (or usage) of CO2. The main methods for capturing CO2 are: post-combustion; pre-combustion; and oxy-fuel combustion. Post-combustion technology separates CO2 from the flue gas, by using a chemical solvent for instance, after the fuel is burnt. Pre-combustion methods involve converting the fuel into a gas mixture consisting of hydrogen and CO2 before it is burnt. Once the CO2 is separated, the remaining hydrogen-rich mixture can be used as fuel. Finally, oxy-fuel technology involves burning a fuel with almost pure oxygen to produce CO2 and steam, with the released CO2 subsequently captured.
Post-combustion and oxy-fuel equipment can be fitted to new plants or retrofitted to existing facilities that were originally built without it. Pre-combustion methods require larger modifications to the operation of the facility and are therefore more suitable to new plants.
Currently operational facilities fitted with CCUS can capture around 90% of the CO2 present in flue gas. It is technologically possible to achieve higher capture rates, and research is ongoing to reduce the costs of doing so. CO2 can also be captured directly from the atmosphere by drawing in air using fans and passing it through an environment consisting of solid sorbents or liquid solvents. This practice is more energy intensive and therefore more expensive as CO2 has a much lower concentration in the atmosphere than in flue gas.
Once the CO2 has been captured, it is compressed into a liquid state and transported by pipeline, ship, rail or road tanker. CO2 can then be injected into deep geological formations, usually at depths of 1 km or more, to be permanently stored in depleted oil and gas reservoirs, coalbeds or deep saline aquifers, where the geology is suitable. Evidence suggests there is more underground storage available globally than is actually needed to meet climate targets and almost every high-emitting nation has already demonstrated having its own substantial storage resource.
An alternative to permanent storage is to use the captured CO2 as an input for commercial products and services, but the climate implications of this are not clear-cut and require careful examination (discussed further below).
What is the potential of CCUS for tackling climate change?
CCUS can play a strategic role in global decarbonisation efforts in a number of ways. These include: (i) reducing emissions in ‘hard-to-abate’ industries (those that are particularly difficult to decarbonise); (ii) producing low-carbon electricity and hydrogen, which can be used to decarbonise various activities; and (iii) removing existing CO2 from the atmosphere. The various roles of CCUS can also help make the energy supply more diverse and flexible, in turn contributing to energy security, which has become a growing priority for governments around the world.
CCUS offers the most cost-effective option in many regions for the deep decarbonisation of a number of hard-to-abate industries, including iron, steel and chemicals. Moreover, CCUS is virtually the only known technological option for achieving deep emissions cuts in cement production, an industry that produces almost 7% of the world’s emissions.
As a way of producing electricity and hydrogen in a low-carbon manner, CCUS can also feed into efforts to switch a wider range of sectors away from fossil fuels. CCUS can be installed on power plants running on coal, gas, biomass or waste. The low-carbon electricity created can then replace fossil fuels as an energy source, including in personal transport, space heating and the extraction of low- and medium-temperature heat in industry. Meanwhile, hydrogen can serve as a more direct substitute for fossil fuels in combustion processes, as feedstock in industrial applications, or in long-haul transport.
Growing evidence indicates that removing carbon dioxide from the atmosphere will be instrumental to reaching net zero emissions globally. The latest IPCC assessment report warns that the deployment of carbon dioxide removal (CDR) technologies is ‘unavoidable’ if net zero emissions are to be achieved. However, CDR should be used to complement, not replace, wider action on carbon mitigation. Two of the main ways of removing CO2 from the atmosphere – bioenergy with carbon capture and storage (BECCS) and direct air carbon capture and storage (DACCS) – both share a technological foundation with CCUS. DACCS enables the capture of CO2 directly from the atmosphere while BECCS can result in CO2 removal on a net basis where the biomass is sustainably sourced.
What is the current state of CCUS development globally?
CCUS development has gained significant momentum in recent years, driven by strengthened climate targets and subsequently increased policy support for the technology around the world. In 2022, 61 new CCUS facilities were added to the project pipeline globally, bringing the global total of CCUS projects to 30 in operation, 11 under construction and 153 in development. The US already has more CCUS projects than any other country, and its landmark Inflation Reduction Act of 2022 is expected to drive further deployment of the technology in the coming years.
In Europe, countries including the UK, Netherlands and Norway are looking to develop CCUS in regional industrial clusters, in which multiple emitters can benefit economically from shared transportation and storage infrastructure.
What is carbon ‘usage’ and where does it fit into efforts to tackle climate change?
Usage (or utilisation) refers to using the captured CO2 to produce commercially marketable products or services. CO2 usage does not necessarily reduce emissions nor deliver a net climate benefit, once indirect and other effects have been accounted for. For example, Enhanced Oil Recovery (EOR), which injects CO2 into oil and gas reservoirs to increase extraction, is one of the most well-known forms of CO2 usage and has been employed for decades. With CCUS increasingly looked to for its decarbonisation potential, the majority of projects currently in construction or advanced development globally are for the dedicated storage of CO2 rather than EOR. While EOR can utilise and store CO2 at scale, it may not yield any net climate benefit, and may even be detrimental when the usage of the oil extracted from the process is considered. In most commercial applications to date – including EOR, fertiliser production, food and beverage production and refrigeration – CO2 is used directly without being chemically altered. Applications that involve converting the CO2 to useful products such as building materials and plastics through chemical and biological processes have yet to be further developed.
It is crucial to assess the climate impact of CO2 usage on a lifecycle basis, considering both the source and the end use of the CO2. For instance, using CO2 captured from a fossil fuel origin would impact the overall ‘carbon budget’ in the atmosphere differently to using CO2 captured directly from the air. Even if we only consider areas of CO2 usage that offer a net climate benefit, the amount of CO2 that needs to be captured through CCUS to deliver large-scale emission reductions is much larger than the current market for CO2 usage. The market is not expected to grow substantially in the short term either. Therefore, while CO2 usage is an important area for further investigation, the immediate focus at least needs to be on securing permanent storage for the captured CO2.
What are the key concerns around CCUS?
High cost is perhaps the most commonly cited drawback of CCUS. CCUS facilities are capital-intensive to deploy and energy-intensive to operate, making them particularly expensive when energy costs are high. There are also risks and uncertainties around the technological performance of CCUS operations. However, given tightening climate targets and increasing carbon prices, reducing emissions is not optional. Therefore, the cost and risks of CCUS should be compared with alternative decarbonisation pathways rather than with ‘doing nothing’. Limiting the availability of CCUS is actually likely to increase reliance on technologies that are currently more expensive and at earlier stages of development. For example, incorporating CO2 capture into steel production raises estimated costs by less than 10%, but approaches based on hydrogen produced from renewables can raise costs by 35–70% compared with conventional production methods.
The cost of CCUS will continue to fall as the market expands and technologies develop. For example, the cost of CO2 capture in power generation reduced by 35% from the first to the second large-scale CCUS facility in that sector. Costs also need to be assessed in terms of wider economic benefits. Notably, CCUS can allow the continued operation of energy-intensive industries in a net zero-compliant way, in turn preventing the many jobs and assets that they support from becoming stranded.
CO2 leakages from storage sites could lead to possible environmental damages and the reversal of intended emissions savings. However, strong regulations for projects’ selection, management and monitoring of storage sites are in place and being developed. Furthermore, many of the potential storage sites being considered are well-understood geological formations that have already stored gas and CO₂ naturally for millions of years, implying that leakage risk overall is relatively small.
This Explainer was updated by Esin Serin in March 2023.